Managing Alaska Soils
FGV-00043 View this publication in PDF form to print or download.
Essential Soil Science Topics for the Commercial and Backyard Crop Producer
Soils are a natural resource with diverse physical, chemical and biological properties that influence their potential uses for agricultural, engineering and environmental practices. Understanding how to use soils to attain maximum benefit while preserving this natural resource is a prime reason for understanding the basic principles of soils.
Contents
- Soil Profile and Horizons
- Soil Components
- Soil Texture
- Soil Structure
- Bulk Density
- Soil pH Soil Buffer pH and Lime Requirements
- Primary Nutrients
- Secondary Nutrients
- Micronutrients
- References
Soil Profile and Horizons
Examining a vertical soil section in a roadside cut or in the walls of a pit reveals the presence of several more or less distinct horizontal layers. This vertical soil section is called a profile; the individual layers are called horizons. A complete agricultural soil profile contains the following horizons:
The “A” horizon is the upper layer of soil that contains organic matter and minerals; it is more biologically active and darker in color than underlying horizons.
The “E” horizon is light in color and is the subsurface layer found directly under the A horizon. It is where maximum leaching, or eluviation, of clay, organic matter, iron and aluminum oxides occurs.
The “B” horizon is the subsurface layer below the E horizon, where materials from layers above accumulate. It usually contains less organic matter and more clay and is brighter in color than the A horizon.
The “C” horizon is less a developed layer and more closely resembles the parent geological materials of soil.
The “R” horizon contains the parent geological materials of the soil.
In general, people such as farmers, gardeners, and environmental engineers are more interested in the A horizon because that is the horizon from which the plant is growing, while engineers for road or house construction are more interested in the B, C or R horizons. In agriculture, only the two zones where plant roots can grow are usually considered: one 0–15 cm (0–6 inches) in depth and a lower one 15– 30 cm (6–12 inches) in depth. However, in Alaska, most plant roots are concentrated in top 0–15 cm.
Soil Components
Mineral soils consist of four major components: organic matter (~5% by volume), mineral particles (~45 percent), air (~25 percent) and water (~25 percent). The impact of one component is seldom independent of the others. Organic matter, by binding mineral particles into aggregates, increases the number of large soil pores, thereby influencing water and air relationships.
Organic Matter
Soil organic matter is made of partially and well-decomposed plant and animal residues plus other organic compounds synthesized by soil microbes as decay occurs. The soil organic matter binds mineral particles into aggregates that are largely responsible for the loose, easily managed condition of productive soils and increases the soil’s water-holding capacity.
Soil organic matter contains nutrients that can be used by plants. These nutrients are released through a process called mineralization, which converts organic forms of nitrogen (N), phosphorus (P) and boron (B) into plant-available forms. Soil organic matter can also bind a variety of metal forms of plant nutrients such as copper (Cu), zinc (Zn) and iron (Fe). If the bond is strong and not soluble in water, plants may not able to use them; however, if the bond is not strong and those nutrients can be released in water, then they are available for plant uptake.
Soil organic matter possesses static charges. The number of charges soil organic matter can hold is totally dependent on soil pH. In soil with a pH of 5 to 6.5, like most Alaska soil, the organic matter holds negative charges, which contribute to the ability of a soil to hold nutrients with positive charges, such as NH4+, Cu2+, Zn2+, Ca2+, Mg2+, etc.
In addition to the original plant and animal residues, soil organic matter contains complex compounds relatively resistant to decay. These materials, which contain living and dead soil microbes as well as residues such as lignin, are referred to as soil humus. Humus is usually black or brown in color and has a high adsorptive capacity to hold water and nutrient ions. Remarkably, a small amount of humus increases the soil’s capacity to promote plant growth through its effect on the chemical, biological and physical properties of the soil.
Organic material in general makes up less than 10 percent of most mineral soils, yet it is extremely important in terms of plant nutrition. Organic matter can react with various soil constituents, and it may have a large influence on soil chemical properties. In addition, because organic matter is composed largely of decayed plant material, it contains plant nutrients that may become available for use by growing plants as the organic matter decomposes. The bulk of several nutrients, including N, P and sulfur (S), may be contained in the organic fraction of a soil. As a result, the available levels of these nutrients are affected by the processes of organic matter decomposition. Organic matter decomposition is dependent upon adequate aeration and moisture and increases with increasing soil temperature. This process is also accelerated when organic matter is incorporated into soil by tillage operations. (See Extension publication FGV-00349, Organic Fertilizers.)
Mineral Materials
Mineral particles are inorganic soil components of different sizes: clay (<0.002 mm), silt (0.002–0.05 mm), sand (0.05–2 mm) and gravel (>2mm). Unlike organic matter, the mineral fraction in soil remains relatively unchanged over time. The properties of minerals are inherited from the parent geological material. If a soil is developed from wind deposition materials, the soil particles will consist predominately of silt, but if it developed from lake deposition materials, the clay fraction will be high. Minerals in soil also undergo a weathering process over time. Under different climatic conditions, soil will develop different properties and colors. Alaska soils are relatively young and less weathered and have rich mineral nutrients such as potassium (K).
Air
Plant roots must have oxygen in order to respire and function normally. Adequate soil aeration is very important in maintaining the best growth and production. Soils with a high proportion of tiny pores tend to be poorly aerated and water dominated. Water content of soil largely determines the content of soil air since the air occupies the soil pores not filled with water. Soils are roughly 50 percent pore space and 50 percent solids. If the gas exchange between the soil and atmosphere is limited by inadequate aeration (for example, if it becomes waterlogged), oxygen is depleted and plants die.
Water
Water is held in the soil pores with varying degrees of tightness, depending on the amount of water present and the size of the pores. Together with its soluble parts, including nutrient elements (e.g., calcium (Ca), K, N and P), soil water makes up the soil solution, which is the critical medium for transporting nutrients to growing plants. An important property of the soil solution is its acidity or alkalinity, i.e., the amount of hydrogen ions (H+) or hydroxide ions (OH–) in the soil solution. Soil solution acidity, or pH, influences the solubility and availability of several essential nutrient elements, such as iron (Fe), manganese (Mn), P, Zn, Cu and Mo, to plants.
The force that holds soil pore water is referred to as soil moisture tension. Small pores possess more power to hold water and vice versa. Soil water held strongly by pores is not available for the plant to use and is referred to as unavailable water. The wilting point occurs when there is not enough water in the soil for the plant to use. The maximum amount of water a soil can hold after gravitational drainage is referred to as field capacity. During and immediately following a heavy rain or irrigation, pores in the upper soil zones are often entirely filled with water. When soil has been saturated, moisture will continue to drain away for 24 to 48 hours, after which the soil is at field capacity. The amount of water in soil that can be used by plant is the plant-available water in soil. To calculate the amount of water available (grams of water per cubic centimeter soil), you need to subtract the water content at wilting point from the soil water content at field capacity. One unit of expression for pore force or tension is the atmosphere (normal atmospheric pressure at sea level), 14.696 lb in2. The metric term “bar” is also used; 1 bar is equal to 14.5 lb in2. Since bar and atmosphere are essentially the same, they can be used interchangeably for most purposes. The greater the amount of water in a given soil, the lower the soil moisture tension. At the same soil moisture tension, a clay soil holds more water than a sandy soil.
Water in excess of the field capacity is termed “gravitational.” Gravitational water is of limited use to plants because it is present in the soil for only a short time. While in the soil, it occupies the larger pores, thereby reducing soil aeration. Removing gravitational water from the soil by draining is generally required for optimum plant growth.
Field capacity (⅓ atmosphere) is considered to be the upper limit of available water for plant use. A soil moisture level where a plant cannot regain turgidity in a saturated atmosphere is called the permanent wilting point (15 atmospheres). This is considered the lower limit of available soil moisture for plant use. The amount of water in a soil at field capacity and permanent wilting point is a function of soil texture.
Soil Texture
Soil texture is determined by the relative amount of various-sized particles that make up a soil. Soil particles are divided into three size categories. The smallest or finest is clay, followed by silt and then sand, the coarsest or largest particle category. The proportion of sand, silt and clay in the mineral or inorganic portion of a soil determines its texture. There are 12 soil textural classes ranging from sand, which is the coarsest class, to clay, which is the finest. Loamy sand, sandy loam, silt loam and clay loam are common soil textural classes with progressively finer particles. The texture of a soil affects its physical properties (how much water it holds, how difficult it is to till, etc.) as well as its chemical properties. In general, a fine-textured soil has more particle surface area than a coursetextured soil per given volume of soil. The surfaces of soil particles are chemically active. Fine-textured soils are more chemically active than coarse-textured soils and are able to hold more nutrients; they also have a greater capacity to bind or “fix” nutrients, rendering them unavailable to the plant.
The percentage of sand, silt and clay may be plotted on the soil textural triangle to determine the textural class of that soil (see Figure 1). For example, a soil containing 13 percent clay, 41 percent silt and 46 percent sand would have a loam texture. Some physical characteristics of the basic textural class are listed below.
Sand
Sand is coarse and single-grained, and the individual grains can be felt or seen. Squeezed between the thumb and the fingers when moist, it will form a cast, but will crumble when touched. A ribbon will not form.
Sandy Loam
A sandy loam contains mostly sand but has enough silt and clay to make it somewhat cohesive. If squeezed when moist, it will form a cast which will withstand careful handling without breaking; however, a ribbon won’t form.
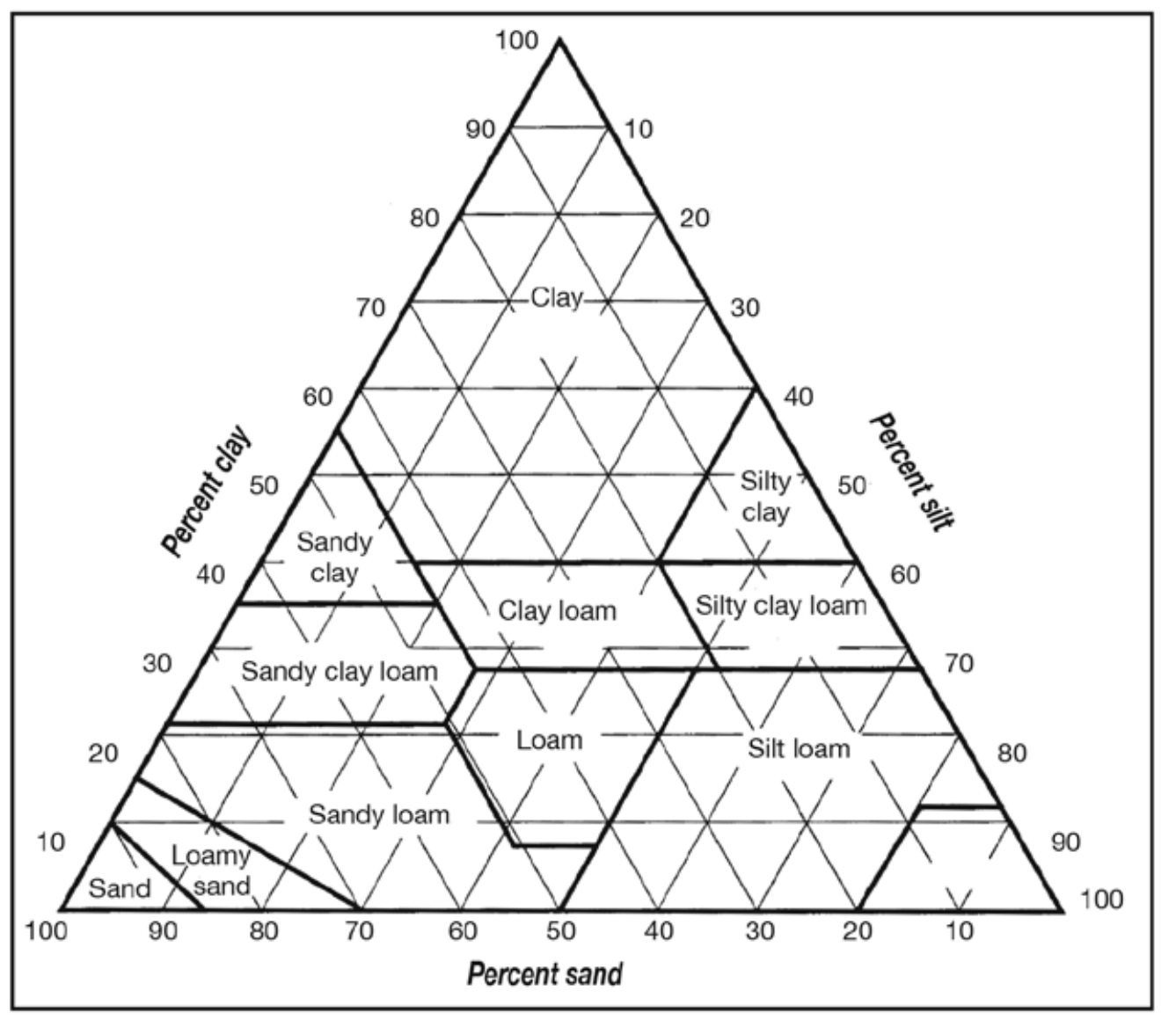
Loam
An ideal loam is a mixture of sand, silt and clay particles that exhibits the properties of those soil separates in about equal proportions. Neither grittiness nor smoothness predominate. A weak ribbon less than 1 inch forms before breaking.
Silt Loam
In a silt loam, over half of the particles are silt size (0.002 to 0.05 mm), with a moderate amount of sand and only a small amount of clay present. When dry and pulverized, it feels soft and floury. When moistened and squeezed between thumb and finger, it will only form a ribbon (less than 1 inch) with a broken appearance that barely sustains its own weight. If moist and kneaded in the hand, it tends to work into a heavy, compact mass.
Clay
A clay soil must contain at least 35 percent of the clay separate, and, in most cases, not less than 40 percent. A clay usually forms very hard lumps or clods when dry, and it is quite plastic and usually sticky when wet. It will form a long, flexible ribbon.
Peat Soil
Peat soil is composed of more than 50 percent organic matter; it is slightly decomposed but highly fibrous, with easily recognizable plant remains.
Muck Soil
Muck soil is an organic soil containing highly decomposed organic matter in which the original plant parts are not recognizable. It contains more mineral matter and is usually darker in color than peat soil.
Soil Structure
Soil texture is important, but by no means does it provide enough information to assess potential soil productivity. Structure is also valuable as it relates to the grouping or arrangement of soil particles. A soil profile may be dominated by a single type of structure. More often, however, several types are encountered in the different horizons. Structure describes the gross, overall combination of the primary soil separates into secondary groupings called aggregates or peds. Soil conditions and characteristics such as water movement, heat transfer, aeration and porosity are influenced by structure. The important physical changes imposed by a farmer on land are structural rather than textural. Texture is a permanent soil property that cannot be altered; structure can be changed by plowing, cultivating, draining, liming or manuring soil. The most common structure for topsoil is granular; other structures such as platy, blocky or prismatic usually occur beneath the topsoil horizon.
Bulk Density
An important soil measurement is bulk density, or the weight of dry soil per unit of bulk volume, including the air space. Silt loams, clays and clay loams generally have lower bulk densities of 1.00 to 1.60 g/cm3. Higher bulk densities occur where essentially no macropores exist, which greatly impairs root growth. Adding crop residue or manure tends to decrease bulk densities of surface soils. In the lower soil profile layers, bulk density is generally higher than in surface horizons, apparently resulting from lower organic matter content, less aggregation and root penetration, and compaction from overlying layers.
Soil pH, Soil Buffer pH and Lime Requirements
Soil pH is a measurement of H+ concentration in a soil solution. It indicates whether a soil is acidic (pH < 7) or alkaline (pH >7). Most plants roots grow well in a slightly acid soil (pH around 6.5). Soil solution pH is relatively stable because it is buffered by soil buffer pH. Soil buffer pH is a measurement of H+ concentration in the exchange site. The amount of H+ in the exchange site is more than the H+ in solution. Therefore, when solution H+ is consumed, H+ in exchange site will supplement H+ in soil solution to compensate solution H+ loss. This is the way that a soil can maintain a relative stable soil pH for microbes and plant roots to live. If soil pH is lower than 5.5, a lime application should be considered. The amount of lime applied is directly related to soil buffer pH. Below is a table for buffer pH and the lime requirement for a target pH of 7 for the top 6 inches of soil in a acre or hectare.
Lime requirement and target pH after lime application
Pure CaCO3 required (tons/acre) to increase soil-water pH to following values
Soil-buffer pH | pH 6.0 | pH 6.4 | pH 6.8 |
---|---|---|---|
6.7 | 1.0 | 1.2 | 1.4 |
6.6 | 1.4 | 1.7 | 1.9 |
6.5 | 1.8 | 2.2 | 2.5 |
6.4 | 2.3 | 2.7 | 3.1 |
6.3 | 2.7 | 3.2 | 3.7 |
6.2 | 3.1 | 3.7 | 4.2 |
6.1 | 3.5 | 4.2 | 4.8 |
6.0 | 3.9 | 4.7 | 5.4 |
5.9 | 4.4 | 5.2 | 6.0 |
5.8 | 4.8 | 5.7 | 6.5 |
5.7 | 5.2 | 6.2 | 7.1 |
5.6 | 5.6 | 6.7 | 7.7 |
5.5 | 6.0 | 7.2 | 8.3 |
5.4 | 6.5 | 7.7 | 8.9 |
5.3 | 6.9 | 8.2 | 9.4 |
Adapted from Frank Sikora, Clemson University
pH – Effects on Alaska Soils
Soil acidity is important because it determines solubility of soil minerals and it affects many microbial processes such as organic matter decomposition and nitrogen fixation. Some soil minerals contain nutrients, and these nutrients may become available for plant growth only when the soil pH is in a favorable range. Additionally, some soil minerals contain elements that are toxic to plants. Primary among these elements is aluminum (Al), which is a major constituent of all mineral soils. Aluminum may be released when soil pH drops below 5.2, and many plants will not grow well when this happens. The general relationship between soil pH and nutrient availability is shown in Figure 2. The widths of the bands represent the relative availability of each nutrient. Note that availability of most of the nutrients is maximized in the pH range of 5.5 to 6.5. This is the optimum pH range for many cultivated plants, although the optimum pH level for various plants may differ widely.
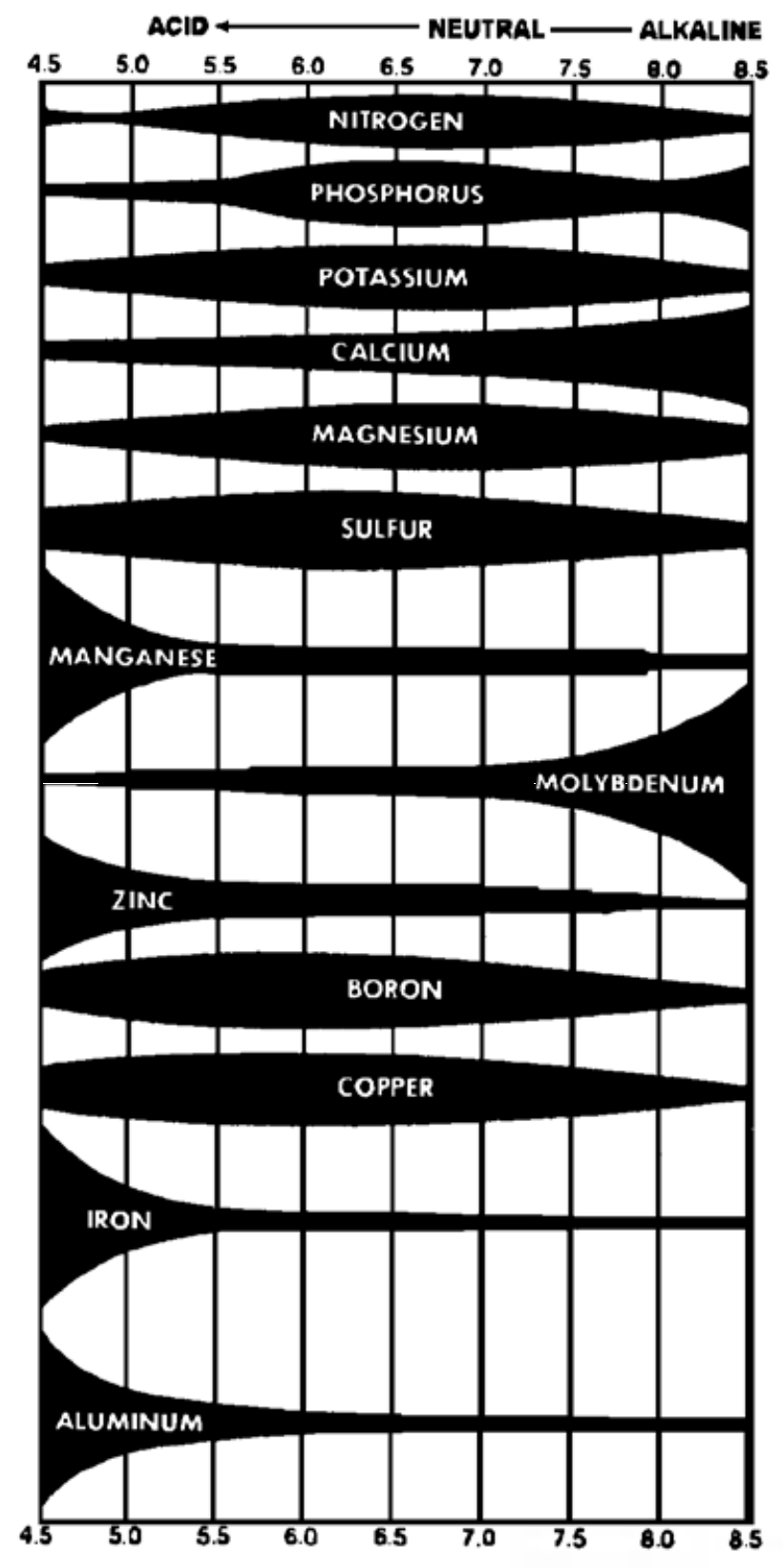
Most soils in Southcentral Alaska, in their unlimed condition, have a pH lower than 7.0 (pH levels as low as 4 are not uncommon). Some soils in the Interior are neutral to slightly alkaline. Soil pH may be adjusted by the addition of liming materials (to raise pH) or acidifying materials (to lower pH). Plants generally increase soil acidity (or decrease soil pH) as they grow. Additionally, many N fertilizer materials add considerable acidity to soils. Therefore, most corrective action is undertaken to raise pH rather than to lower it. Liming materials are generally calcium oxides, hydroxides or carbonates, which combine with H+ ions to decrease acidity. All are adequate for liming agricultural soils.
Most commercial lime is simply crushed limestone and may be either dolomitic or calcitic. Dolomitic lime is a mix of Ca and Mg carbonates, while calcitic lime refers to Ca carbonates. Application of dolomitic lime may be desirable where the Mg supply is insufficient for crop production.
Since lime is fairly slow to react in soil, recommended amounts should be applied as early as convenient; lime may be applied one to two years before needed for a crop to ensure optimum reaction time. However, some benefit is realized in the year of application. Hydrated lime or quick lime will react more rapidly than crushed limestone. One of the best methods of application is to broadcast the lime and thoroughly mix it with the soil during tilling. The better the soil contact with the lime and the finer the lime particles, the quicker soil acidity will be neutralized. (See Extension publication FGV-00348, Field Crop Fertilizer Recommendations for Alaska: Fertilizer Nutrient Sources and Lime.)
Soils may be acidified by adding elemental S or by direct application of acidic materials such as sulfuric acid (H2SO4), aluminum sulfate (Al2(SO4)3) or ferrous sulfate (FeSO4). Elemental S produces acidity as it is converted to sulfuric acid by soil microbes. The microbial transformation to sulfuric acid is slow, and elemental S should be applied well in advance of the growing crop. Extreme care should be taken when acidifying soils since it is easy to reduce soil pH to undesirable levels.
Electrical Conductivity
Electric conductivity is a measurement of soluble salt in soil solution. Salts can accumulate from the weathering of rocks and minerals, rainfall, ground waters and irrigation. Once deposited or released into the soil, the salts can be brought to the surface by the upward movement of water, which evaporates, leaving the salts behind. The salts are primarily chlorides and sulfates of Ca, Mg, Na and K. High levels of these salts cannot be tolerated by most crops.
Electrical conductivity (EC) can give an indication of the salt levels. This is determined by measuring the ability of saturated soil to conduct electricity. The more salt in a solution, the higher the EC. Electrical conductivity is expressed as millimhos per centimeter (mmho/cm) or decisiemens per meter (ds/m); 1 ds/m = 1 mmho/cm. Most crops perform best if the EC is below 1.5 mmho/cm, although salinity tolerance varies with crops. If a soil has an EC of more than 4, crops will have serious growth problems. Improper irrigation (i.e., not enough water) usually is the major cause for salt accumulation in surface soil.
Cation Exchange Capacity
Cation exchange capacity (CEC) is a measurement of the static negative charges that soil particles have. Soil colloids with negative charges attract and hold cations (e.g., Ca2+, Mg2+, K+, Na+, A13+, NH4+, H+). Humus particles also possess negative charges, but the number varies depending on soil pH. In general, an acidic pH decreases the negative charges, and an alkaline pH promotes negative charges for humus. Therefore, soil CEC is a contribution of both soil humus and minerals. Clay and humus account for most of the CEC, but finer fractions of silt can have a limited number of exchange sites. The CEC is expressed as milliequivalents (meq) of positive charge that can be held (adsorbed) by 100 grams of soil (oven-dry basis) or as centimoles of positive charge per kilogram of soil (cmol/kg); 1 meq/100g = 1 cmol/kg. Sandy soils have a lower CEC than clay soils because the coarse-textured soils are commonly lower in clay and humus content. The texture and organic matter of a soil influence the CEC. The CEC of a loam or silt loam soil could range from 10 to 25 meq/100g.
All higher plants require at least 17 nutrient elements for proper development. Of these, three (carbon, hydrogen and oxygen) are provided by air and water, and the remaining 14 are usually provided by the soil. Six of the 14 elements normally provided by soil are required by growing plants in relatively large amounts and include N, P, K, Ca, Mg and S. The first three (N, P and K) are called primary plant nutrients because they are most commonly limiting in agricultural situations. A complete fertilizer contains the three primary nutrients.
The others, Ca, Mg and S, are just as important for normal plant growth but are called secondary nutrients because they are less frequently limiting to crop production than the primary nutrients. The remaining plant nutrients, B, chlorine (Cl), Cu, Fe, Mn, Mo, nickel (Ni) and Zn are required in relatively small amounts and are called micronutrients. Plants may contain levels of some micronutrients as high as the primary and secondary nutrients, but the minimum required micronutrient level is generally much lower. Again, these nutrients are just as critical to normal plant growth as the primary and secondary nutrients.
Primary Nutrients
Nitrogen (N)
Nitrogen is one of the more difficult nutrients to analyze. This is because a major portion of soil N may be contained in the soil organic matter. Availability of organic N is dependent on breakdown of organic matter, which is difficult to estimate. Without knowing what soil temperature and moisture are going to be in the future, a prediction of N release from organic matter is no more than a guess. The amount of inorganic N (i.e., NH4+ and NO3–) in soils is fairly easy to determine. However, it may be quickly converted between N forms and is easily lost from the rooting zone of the soil. There is no way to relate inorganic N present in a fall sampling to that which may be present in the spring. An accurate determination of the N available to plants at the time of soil sampling can be made, although even this may not provide reliable information concerning an entire growing season. If soil N analysis is to be used for making fertilizer recommendations, it is important that the sampling be done either shortly before planting time or during the growing season.
A soils laboratory can analyze soil for either total or inorganic N. The total N analysis is not particularly useful for agricultural purposes. Inorganic N analysis provides a measure of N at the time of sampling, but remember, if a soil is over-irrigated or if a heavy rainfall occurs, much of this N can be lost, and a reevaluation of N status may be required.
Nitrogen is generally required in larger amounts than any other plant nutrient. It may be taken up in two forms, nitrate and ammonium. Nitrate (NO3–) is an anion, or negatively charged molecule, while ammonium (NH4+) is a cation, or positively charged molecule. Because of their charges, these two forms of N behave very differently. Soil particles and soil organic matter generally have a negative charge; therefore, they attract positively charged cations and repel negatively charged anions, much like the positive and negative responses observed when two magnets are put together. Nitrate (negative charge), which is not held by soils, is easily lost through leaching, while positively charged ammonium is not. When ammonium fertilizers are applied, soil bacteria can convert the N to nitrate in a process called “nitrification.” The rate at which nitrification takes place is largely dependent on soil temperature, and nitrification occurs mainly in the summer months. In waterlogged soils, nitrate may be converted to several gaseous forms of N and lost to the atmosphere. To avoid N loss due to gaseous loss or leaching, N-containing fertilizers should not be applied in the fall. Most plants are not particular about the form of N they take up. However, nitrate-based fertilizers are preferred for hay/pasture field because they can easily move downward to the root zone to avoid ammonia volatilization (NH4+ being converted to NH3 and escaped in gas form). Too much ammonium in the root zone is harmful to plants.
Roughly 95 percent of soil N is found in the organic material in unfertilized soils. In these situations, organic matter decomposition is critical to N nutrition of plants. Nitrogen applied in inorganic fertilizers is generally very soluble and may be subject to loss by leaching if excessive rain or irrigation is present. However, N moves to plant roots largely through the flow of water. Therefore, providing adequate water for crop plants is critical to maintaining a satisfactory N supply.
Phosphorus (P)
Phosphorus is tightly bound to soil particles in many soils. The amount removed during soil extraction is very dependent on soil type and on the specific extractant used. The extractant currently recommended for soil analysis of P is called Mehlich-3 extractant, and it is the best for a wide range of conditions. (See UAF AFES Circular 81, Relating Mehlich-3 extractable P to Morgan and Bray 1 extractable P for Alaska Soils.) However, the optimum P soil test value varies substantially in Alaska soils, requiring separate interpretation for each soil type. Phosphorus analysis is one of the most reliable and useful soil tests.
In contrast to N, P is very insoluble in soil systems. Maintaining proper soil pH is critical to providing adequate P for optimum plant growth. When soil pH drops below 5.0, aluminum becomes soluble and will bind P, making it less available for plant use. Alternatively, if soil pH is above 7.5, Ca may bind P, rendering it less available to crops. Within the range of pH 5.0 to 7.5, P solubility is maximized, but it is still low compared to the other primary and secondary nutrients.
Some types of soil minerals have a very strong affinity for P molecules. Primary among these are volcanic ash particles, which predominate in many Alaska soils, particularly in the Kenai and Susitna Valley areas. In these soils, large amounts of fertilizer may be necessary to provide adequate P. Organic matter decreases the ability of soil mineral particles to fix or bind P, so adding organic materials may reduce the P requirement of volcanic ash soils. Another method of reducing the P requirement in P-fixing soils is to apply P fertilizer in a concentrated band to minimize its contact with soil mineral particles and thus decrease fixation.
Potassium (K)
Although the total amount of K in most soils is quite large, a relatively small portion is generally available for plant growth. Most K is held as part of the soil minerals structure or inside layered clay particles, and it may become available very slowly. The release of K from K-bearing minerals is usually not sufficient for crop plants.
Potassium is held tightly enough to soil particles that K fertilizer may be applied in the fall or spring in all but the sandiest soils. In very sandy soils, K fertilizers should be applied only in the spring.
Potassium availability is not strongly affected by soil pH. However, K uptake by plants can be limited if soil moisture is insufficient. Potassium management consists largely of maintaining soil K levels and providing adequate water to the growing crop. A large amount of K can be taken up by crop plants, and this must be replaced by inorganic or organic fertilizers.
Secondary Nutrients
Calcium (Ca)
Calcium is the dominant positively charged cation in nearly all soil systems except those with a very low pH. In soils with a pH above about 4.8, Ca is usually present in amounts adequate for crop growth. In acid soils, however, Ca is subject to leaching, and native Ca levels may be low. This situation can be corrected by using a liming material.
Even when soil Ca levels are satisfactory, supply to growing plants may be interrupted if adequate water is not provided. Calcium moves with water in a plant, and if water flow is interrupted during the formation of certain plant tissues, localized Ca deficiencies may occur. This can result in disorders such as tomato blossom end rot, tip burn in lettuce, cabbage and strawberries, and bitter pit of apples. These and related disorders are more often the result of drought and other plant stresses than of inadequate soil Ca availability.
Magnesium (Mg)
Magnesium is positively charged and, like Ca, is most likely to be deficient in low-pH soils. Under acidic conditions, Mg is very soluble and may be lost due to leaching. If an acidic soil is limed with a material that contains little or no Mg, a deficiency of this nutrient may result. When liming a very acid soil (below pH 5.2), it is a good practice to use a liming material that contains Mg or to monitor soil Mg levels closely.
Magnesium can be added to fertilizers as well as liming materials. Using a Mg fertilizer may be desirable where inexpensive sources of calcitic limes are available or where the soil pH level is adequate. Magnesium is held tightly by negatively charged soil particles and can be applied at any time of the year.
The analyses for available K, Ca and Mg are quite reliable. For Alaska soil, the Mehlich-3 extractant is recommended, although other extractants also produce acceptable results. The main difficulty that may be encountered is when Ca or Mg analyses are conducted on soils with undissolved Ca– or Mg-containing materials. This may be the case if a coarse liming material has recently been applied. In these situations, the analytical results may indicate a higher level of plant-available nutrient than actually exists. This is not usually a problem, and K, Ca and Mg tests usually provide excellent estimates of plant available levels of these nutrients.
Sulfur (S)
Sulfur is taken up by plants as the negatively charged sulfate (SO42–) molecule. Because it is a negatively charged molecule, or anion, sulfate may be easily leached from the soil. Most S, however, is not present in soil in the anionic form but is tied up in soil organic matter. Sulfur availability, therefore, is controlled largely by the amount and rate of organic matter decomposition. In most soils, adequate S for crop plants is supplied through this process and through rainfall.
In soils with a marginal S supply, insufficient S may be taken up by crops to induce S deficiencies. Crops with pungent sap (onions, cabbage, broccoli) generally have high S requirements. Sulfur may be supplied in potassium sulfate or many complete fertilizers or may be applied in materials like gypsum (CaSO4) or elemental S. If elemental S is used, it must be converted to the sulfate anion before being used by the plant. This conversion process produces considerable acidity. Elemental S is used as a soil acidifying agent more often than as a S fertilizer source.
Micronutrients
Several micronutrients, including Mn, Zn, Fe and Cu, behave similarly. As soil pH increases, the solubility of these micronutrients decreases. Therefore, deficiencies of these nutrients are most common in high-pH soils.
Even when plants exhibit deficiencies of these four nutrients, they are usually present in the soil in substantial quantities. However, they are unavailable for plant growth because of unfavorable soil conditions, usually high soil pH. Adding fertilizer may not correct the deficiency in high-pH soil because added nutrients will quickly become unavailable as a result of the soil’s condition. There are two ways to address this problem. One way is to correct the underlying problem, i.e., acidify the soil if it is too alkaline. The other is to add the nutrient in a “chelated” form, which is more readily available to plants. Chelated micronutrients are complexed with a material that increases the solubility of the nutrients, which reduces the degree of fixation by soil minerals and organic matter. In addition, all micronutrients can be applied as foliar sprays. This is a very effective way of applying micronutrients to growing crops, but it does not address the soil problem. Deficiencies will likely occur in subsequent crops.
Manganese (Mn)
In addition to the effects of soil pH described above, Mn solubility is affected by soil water content. Under waterlogged conditions Mn becomes very soluble and can reach toxic levels. This is most likely to occur in acidic soils with pH levels less than 5.5, although it can happen even when soils are not very acidic. Manganese toxicity can also occur when soils are steam-sterilized, and it is a frequent problem in greenhouse production.
Well-drained, high-pH soils are most prone to Mn deficiency. Manganese deficiency can be induced when acidic, low-lying sandy soils are limed to pH levels above 6.5, or when wet, sandy soils are drained.
Zinc (Zn)
Soil acidity is the primary factor affecting Zn availability. Zinc deficiency occurs in moderate- to high-pH soils and may be more pronounced if soil P levels are high. Zinc deficiency may occur in soils with a pH of 6.0 to 7.0 if they are overfertilized with P. Zinc deficiency is most likely to be found in sandy or high-organic matter soils.
Iron (Fe)
Iron deficiencies occur only in high-pH soils. In soils with high pH, most of the Fe is insoluble and therefore unavailable to plants. Reducing the pH with elemental S or some other acidifying agent will correct the problem by solubilizing the Fe in the soil.
Copper (Cu)
Copper solubility also decreases as pH levels increase. Therefore, Cu deficiency can occur in soils with pH levels above 7.5. In contrast to Mn, Zn and Fe, Cu is tightly bound by soil organic matter. As soil organic matter content increases, Cu availability decreases. In some soils with large amounts of organic matter, Cu can be deficient when soil pH is 5.5 or below.
Molybdenum (Mo)
Higher plants require Mo in extremely small amounts. Molybdenum behaves very differently from most other micronutrients. The most common form of soil Mo is anionic, which can be easily leached from sandy soils. Molybdenum is most soluble at high pH levels and is most likely to be deficient in acidic sandy soils. However, Mo deficiencies are sometimes found in moderate-pH, fine-textured soils. This generally occurs where the parent material is low in Mo. Molybdenum is essential to N fixation by legumes, which are very sensitive to Mo deficiencies. The crucifers (broccoli, Brussels sprouts, cauliflower, canola and the forage rapes) all have a high Mo requirement. These plants are sensitive to low Mo levels and also remove substantial quantities of Mo from the soil when harvested.
Boron (B)
Boron is present in soils as an uncharged molecule that is held weakly by various mineral and organic soil constituents; it can be easily leached, particularly from sandy soils. Boron availability is affected by soil pH. It may become unavailable to plants when soil pH is above 6.5.
The crucifers and most of the legumes have high B requirements. The difference between deficient and toxic levels of B is small. Many crops, including cowpeas, lupines, onions and strawberries, are susceptible to B toxicity. Over-application of B fertilizer (>2.5 kg/ha) can be toxic to plant.
Chlorine (Cl)
Chlorine is rarely deficient in agricultural soils. In fact, there are more problems with excess Cl in saline soils than with deficiencies. The functions of Cl are poorly understood, but it appears that Cl is involved in plant tolerance to both cold and drought. It also suppresses plant diseases and prevents physiological leaf spots in cereal crops. Chlorine deficiencies may also be involved in disease susceptibility (for example, in take-all root rot of wheat in the Pacific Northwest). Adequate Cl is provided by rainfall, air pollution and various fertilizer materials, in most situations.
References
Brady, N. C. 1990. The Nature and Properties of Soils. New York: MacMillan Publishing Co.
Follett, R. H., L. S. Murphy, and R. L. Donahue. 1981. Fertilizers and Soil Amendments. Englewood Cliffs, New Jersey: Prentice-Hall, Inc.
Halfacre, G. R. and J. A. Barden. 1979. Horticulture. New York: McGraw-Hill, Inc.
McDole, R. M. Soils Handbook. University of Idaho: College of Agriculture, Cooperative Extension Service and Agricultural Experiment Station.
This publication replaces the following Extension publications: Soil Fundamentals by C. L. Johnson, former Extension Assistant, Palmer Research Station, University of Alaska Fairbanks and Soil Fertility Basics and Soil Sampling and Analysis by J.L. Walworth, former Soil Scientist, Agricultural and Forestry Experiment Station, Palmer Research Center, University of Alaska Fairbanks.
Mingchu Zhang, Professor of Agronomy, Institute of Agriculture, Natural Resources and Extension. Originally compiled by Thomas Jahns, former Agriculture and Horticulture Agent
Revised June 2021