Managing Irrigation for High-Value Crops
FGV-00649 View this publication in PDF form to print or download.
Why Irrigate?
Even in years with above average rainfall, crop growth and yields in many parts of Alaska can still be limited by inadequate water in the soil (soil water) at critical times during the growing season. Short-term periods of soil-water deficit occur in most years. In Southcentral Alaska, for example, rainfall is typically low during the early part of the growing season. Extended periods of drought are less frequent, but they have severe effects when they do occur.
Supplementing rainfall with water supplied by irrigation systems during periods of soil-water deficit not only can increase yields, but can also improve the quality of harvested plant products. This is especially important for high-value crops like vegetables, fruits and ornamentals, where reductions in quality can result in reduced prices or even the complete unsuitability of a crop for market.
Agronomic crops, such as small grain and hay, can also benefit from irrigation, but potential returns may not be enough to justify the cost. The economics of irrigation also depend on markets — high-quality hay markets provide premium prices in some hay growing areas.
Depending on the crop, adequate soil water is most critical during seed germination, seedling or transplant establishment, flowering, fruit set and fruit enlargement. A fluctuating water supply (alternating wet/dry conditions) promotes the development of water-related physiological disorders such as blossom end rot, tipburn and cracking or splitting of fruit.
The objectives of this publication are to (1) give an overview of irrigation systems and important principles of irrigation scheduling and (2) discuss the available tools for monitoring soil-water content, which is central to effective irrigation management.
Irrigation Systems
Irrigation requires a dependable and affordable water supply, sufficient labor for operation and maintenance, an economically responsive crop and the time and skill to manage the system efficiently. Overhead (sprinkler) and drip (trickle) irrigation systems are both commonly used in high-value crop production. There are many variations of each of these application methods, and the best type for an individual grower depends upon many factors: cropping system and rotations, soil type and topography, available capital, equipment and labor, quantity and quality of water, power requirements and total area irrigated, etc.
In the last 25 years, drip and other microirrigation methods (e.g., microsprinklers) have become increasingly popular. Advantages of drip systems include reductions in water use, limited labor requirements after installation, lower pumping costs, reduced incidence of foliar diseases, ease of applying fertilizer and other chemicals to the soil, and the ability to irrigate without conflicting with spraying, harvesting and other field operations. For more information on drip irrigation, see the Extension publication Trickle Irrigation for Alaska Gardens (FGV-00648) and the reference list at the end of this bulletin.
A major disadvantage of drip irrigation, compared to overhead irrigation, is the need for high-quality water and filtration to prevent clogging of emitters. Equipment for overhead irrigation is also good for many years, while many of the components of a drip system must be replaced on an annual basis. In addition to the replacement cost, there is an annual installation cost.
Irrigation Scheduling
Irrigation can profitably increase high-value crop production, but in addition to the necessary capital investment, a commitment of time to manage and monitor the system is required to obtain its full benefit.
Irrigation scheduling refers to methods of determining both the optimum timing and the optimum amount of water to apply. Rainfall, temperature, wind, available water-holding capacity of the soil, soil depth, crop rooting pattern, plant growth rate and stage of crop development all interact to determine when and how much water to apply. The situation is complex, but some reasonably straight forward methods of irrigation scheduling have been developed. The key is to set aside the time during the busy part of the growing season to do the necessary monitoring, record keeping and management that are required.
Ideally, irrigation is timed to deliver water as soon as insufficient water availability and water uptake limit plant growth. Direct measurement of plant-water status is difficult and expensive, so soil-water monitoring methods and water-budget methods are the most commonly used tools for scheduling irrigation. They are frequently used in combination, although many growers find it less time consuming and more practical to rely only on monitoring soil-water content. This has also become more accurate with modern sensors that measure water content on a volume basis. Accurate measurements combined with personal experience and knowledge of your particular soils and cropping system are the foundation of effective irrigation management.
The allowable depletion of available soil water (soil-water deficit) before irrigating varies with soil type, crop growth stage and the type of irrigation used. Traditionally, the general guideline was to allow soil water to drop to about 50 percent of field capacity, then apply enough water to completely refill the root zone of the crop. However, this simple rule of thumb has changed significantly and is inadequate for intensively managed, high-value crops.
For many crops, the best growth response to irrigation is generally achieved by maintaining soil water slightly below field capacity. Current recommendations are to water when the soil-water content is 70–90 percent of field capacity. Drip irrigation makes it possible and practical to maintain soil water in this narrow range because it is easy to make smaller, more frequent water applications.
Soil-Water Monitoring
Methods of monitoring soil water include (1) tensiometers, (2) electrical resistance blocks and (3) modern electronic probes. Tensiometers are the most commonly used and are discussed in the most detail below.
Tensiometers and Resistance Blocks
Measurement of soil-water content is done indirectly with tensiometers or electrical resistance blocks placed at several depths in the plant root zone. Tensiometers are plastic tubes with porous ceramic tips on one end and a vacuum gauge on the other end. Tubes are different lengths to permit placing the porous tip at different depths in the soil. Tensiometers are filled with water, so as the soil dries, water is pulled out through the porous tip. The strength of this suction is measured by the vacuum gauge in centibars (cb), which increases as the soil becomes drier; the measure of dryness is proportional to the water content of the soil.
Field capacity refers to the amount of water a soil can hold against the downward force of gravity. It is the water content remaining in the soil about 2–3 days after a saturating rain or irrigation, when free drainage has practically ceased.
Resistance blocks obtain a similar measure of dryness using the principal of increasing resistance to flow of an electrical current as soil dries. They are usually read with a meter calibrated to convert resistance to centibars. Tensiometers are the most commonly used of these two tools, but resistance blocks have a wider useful range of measurement accuracy that can be more effective on heavy clay soils. Tensiometers also lose their vacuum if the soil becomes too dry. Regular inspection and reestablishment of continuous suction between the porous tip and surrounding soil water is required.
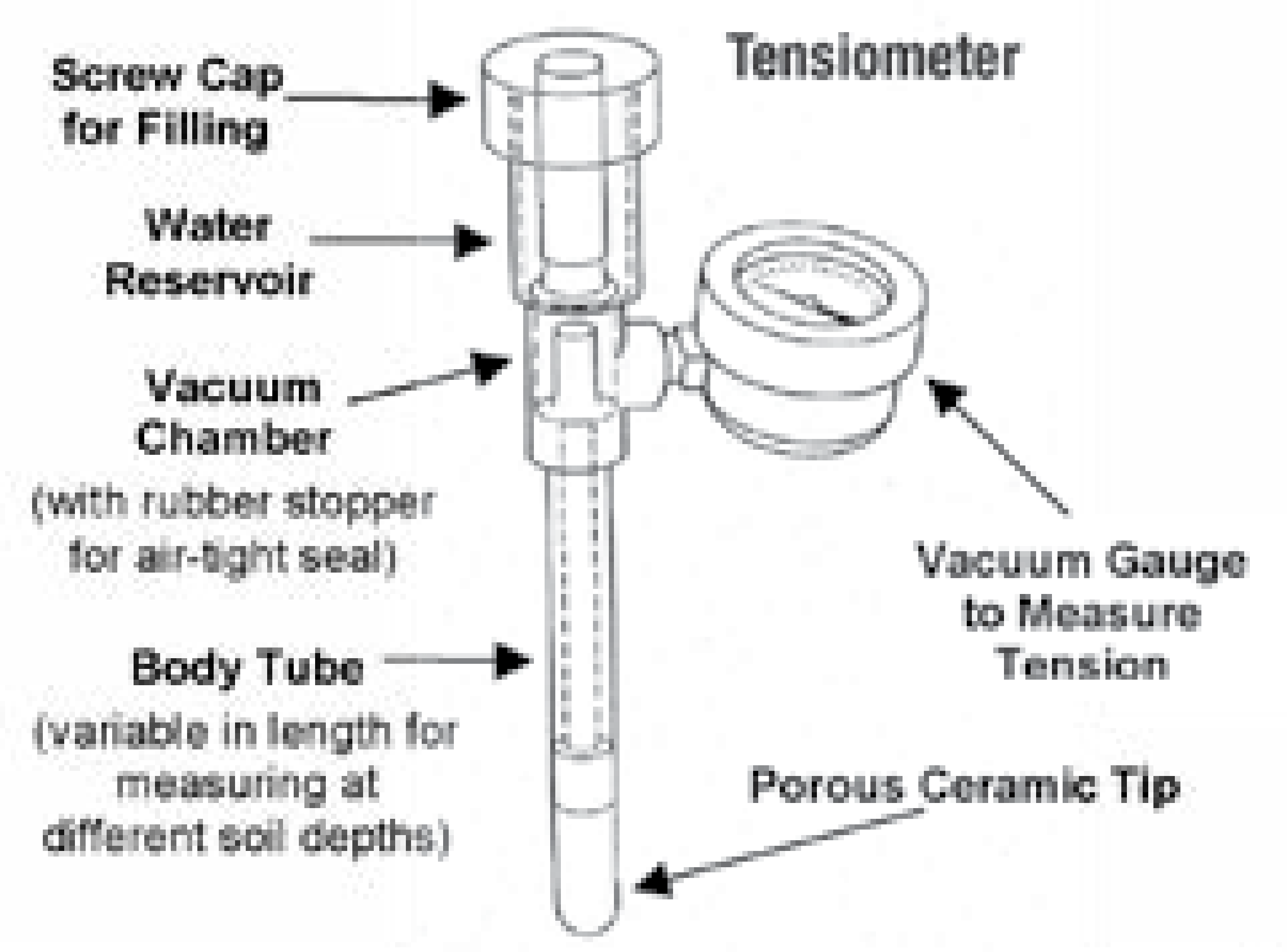
Tensiometers or resistance blocks are used in pairs at different soil depths. One is placed in the zone of most active root uptake, about 6–12 inches for many crops, and the second is set about 8–16 inches below the first (again varying with the rooting pattern of the crop, as modified by the particular soil it is growing in). In simple, approximate terms, the upper instrument is used to determine when to water, and the deeper one tells how much to water.
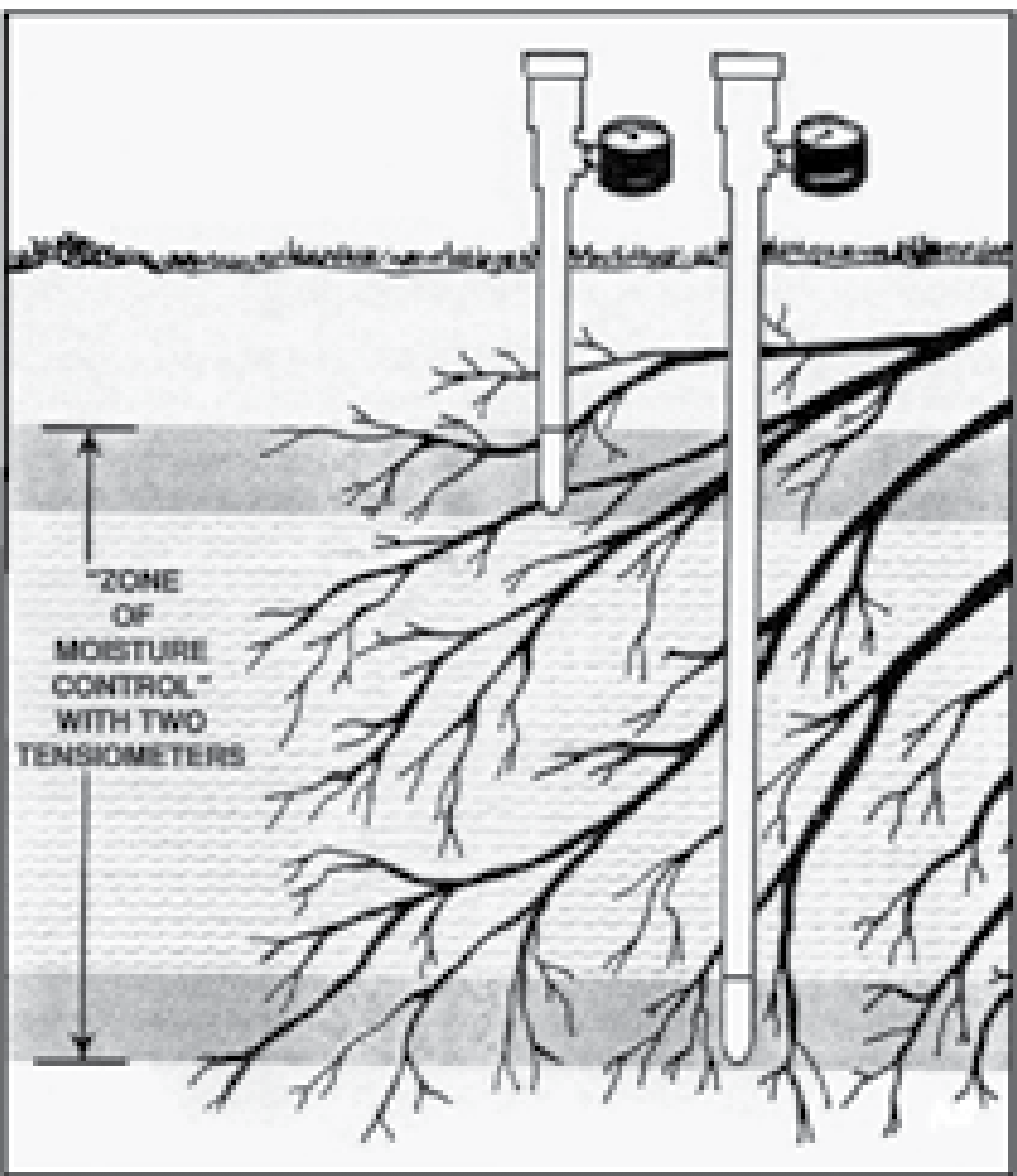
A reading of 0–10 cb tells you the soil is saturated or close to saturation. Field capacity varies with soil type but is about 10–20 cb. Readings 20–40 cb above field capacity measure a soil-water content of about 70–90 percent, so for many soils, crops should be irrigated at tensiometer readings of 20–40 cb. For high-value crops, a tensiometer reading of 25–30 cb is a reasonable value for beginning irrigation on many soils. The amount of water applied should lower the reading of the deep tensiometer to field capacity or less. If it doesn’t, not enough water was applied. If the deep tensiometer reading remains in the saturation zone or below field capacity levels (less than 10–15 cb) for several days after irrigation, too much water was applied.
The 25–30 cb trigger for irrigation is a good general rule, but experience with soils and plant growing systems should be used to refine this guideline. When interpreting tensiometer values, it is important to remember that clay soils hold more water than sandy soils. At the same tensiometer reading, a lighter-textured soil is drier than a heavier-textured one. Clay soils also hold water more tightly—they have more water in them that is unavailable to plant roots.
Electronic Methods
Modern electronic methods of monitoring soil-water content use techniques that measure various electrical properties of water. For example, time domain reflectometry (TDR) measures the dielectric constant of the soil-water media and frequency domain reflectometry (FDR) measures the soil capacitance. TDR and FDR probes are the most commonly used electronic methods of monitoring soil-water content.
Interpreting tensiometer readings (or resistance blocks calibrated to read in the same units) is sometimes confusing because it seems intuitive that larger amounts of water in the soil should give higher readings. Remember, however, that the readings are a measure of soil suction: so higher is drier and lower is wetter.
A major advantage of electronic methods is that they are calibrated to give an actual measurement of soil-water content (volume of water per unit volume of soil). If the desired water content is known, you know exactly how much water to apply to get to that level. Electronic probes also permit the installation of multiple sensors on the same probe, which means that soil-water content can be measured at more than one depth—in the same location—at the same time. The signals from electronic probes also extend out into the soil from each sensor. Their zone of measurement is a sphere that is a much larger volume of soil than the measurement points of tensiometers and resistance blocks.
In combination with computer software programs, these probes can draw a complete picture of the water content in the soil profile. They can be programmed to take measurements at various time intervals, allowing changes to be tracked in moisture content over time. They have the capacity for downloading data from a remote computer, such as an office computer, to determine what areas need to be irrigated before going out in the field.
Electronic methods require an electrical power supply in the field, which is often a combination of batteries and solar panels. Power is necessary to send and receive the electronic measurement signal and to run the datalogger. The datalogger collects and stores measurements as they are made.
These instruments are more complex to install and use than traditional methods of soil-water monitoring, but they also have the potential to give more complete information on soil-water content and facilitate more effective irrigation management.
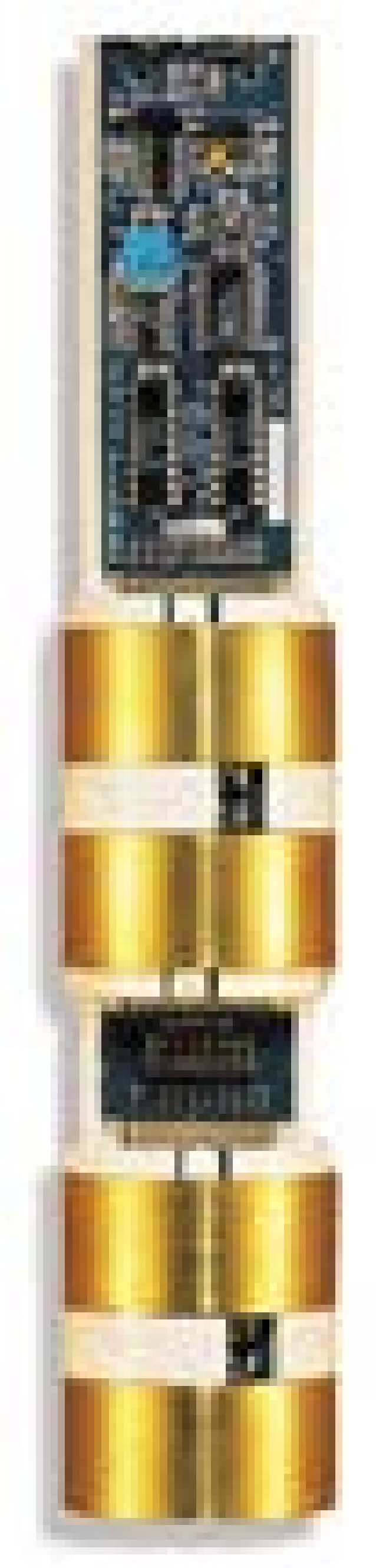
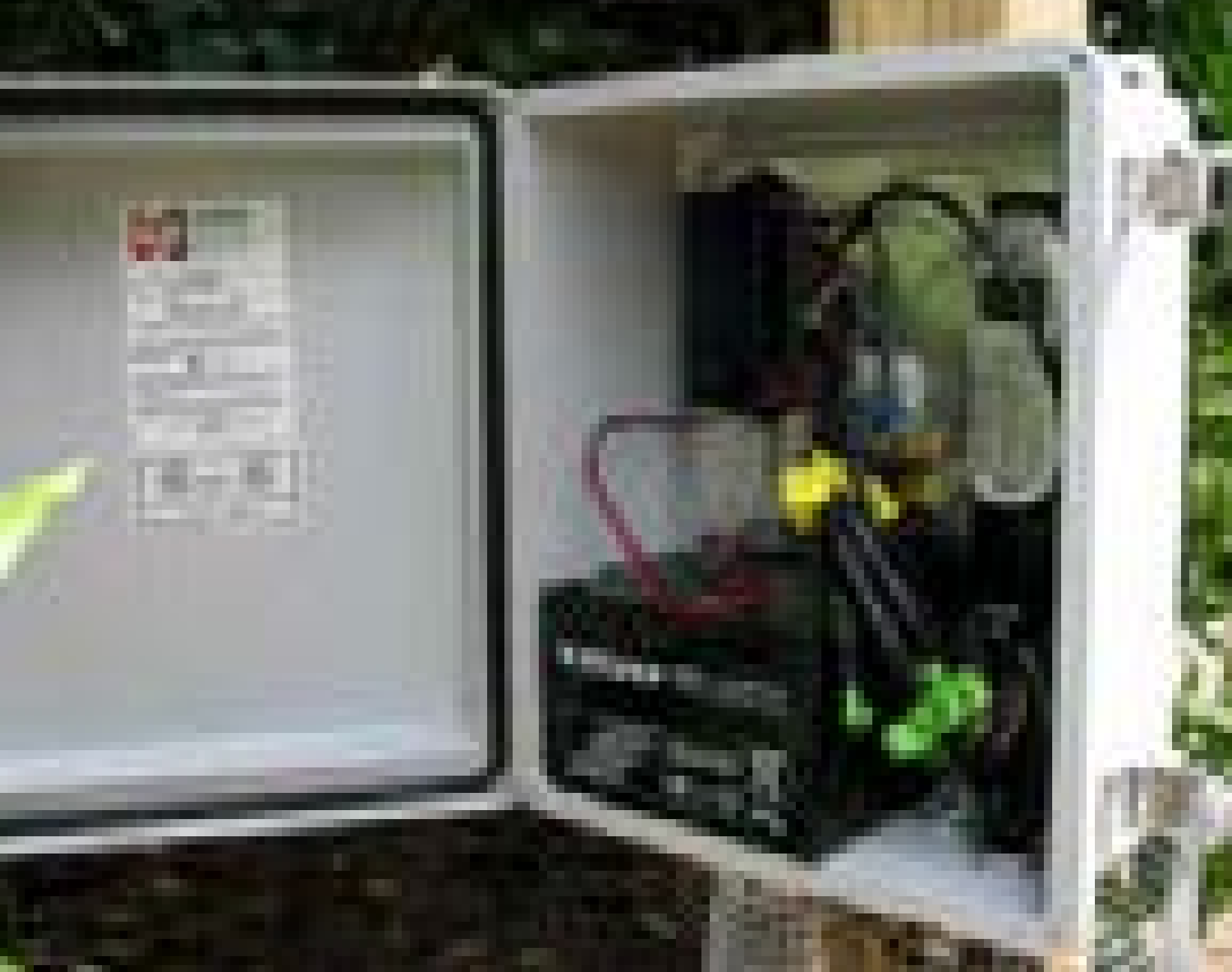
Water Budgets
Water budgets (also called water-balance or checkbook methods) track crop water use and help a grower decide when and how much water to apply. They are based on evapotranspiration (ET) calculations or estimates. Evapotranspiration is the combination of the amount of water evaporated from the soil surface plus the amount of water passing through or transpired by growing plants.
A water budget begins with knowing the current water content of the soil profile compared to the total amount of water the soil can hold or store. The difference between these two values is the soil-water deficit or balance. Water gains (rain or irrigation) and losses (ET) are recorded each day and the water deficit recalculated (the checkbook is “balanced”). When the water deficit (water “withdrawal”) reaches a set value, the crop is irrigated (a “deposit” is made). This set value (allowable soil-water deficit or soil-water depletion) is the same irrigation trigger of about 10–30 percent that was suggested in the section on soil-water monitoring.
Rainfall and irrigation amounts are relatively easy to measure, and the accuracy of water budgets mainly depends upon accurate values for ET. There are many ways to calculate ET, ranging from simple approximations to more sophisticated estimates requiring extensive weather measurements. Many methods produce reasonable values for estimating actual crop water use and irrigation requirements. They can be effectively used in conjunction with the soil-water monitoring methods described previously.
Growers who rely primarily on water budgets to schedule irrigation require accurate ET estimates. ET varies with environmental factors, such as the amount of sunshine, temperature, humidity and wind. Estimates of ET calculated from local weather statistics, or based on evaporation pan measurements, are not readily available in Alaska in a timely manner for practical day-to-day irrigation management.
In addition, to use ET estimates a correction must also be made for the type of crop (since different crops have different water requirements) and the crop growth stage (since water use differs with crop maturity). Accurate values of these crop coefficients are not well known for Alaska growing conditions, which is another problem with using water-budget methods in Alaska.
Summary
Irrigation management contributes to productive and profitable production of high-value crops. There are many different types of irrigation systems, but the principles of effective irrigation management and scheduling are the same no matter how you apply the water. The key to scheduling is accurately determining the amount of water in the soil. The most common monitoring tools are tensiometers, resistance blocks and electronic probes. Tracking soil-water content helps decide both when to irrigate and how much water to apply at each irrigation.
Many factors affect crop-water use, but both yield and quality can be improved with efficient irrigation management and effective irrigation scheduling. By the time you can visually see that a crop needs water, damage has already occurred. Monitoring soil water helps anticipate crop-water needs rather than react to crop-water stress.
For More Information
Trickle Irrigation for Alaska Gardens, FGV-00648. UAF Cooperative Extension Service.
Using Plasticulture Technology for the Intensive Production of Vegetable Crops. 1994. American Society for Horticultural Science. Alexandria, VA.
Drip Irrigation for Row Crops. 1997. Water Management Series Publication 3376. Division of Agriculture and Natural Resources. University of California.
Micro-irrigation of Trees and Vines: A Handbook for Water Managers. 1996. Water Management Series Publication 3378. Division of Agriculture and Natural Resources. University of California.
Scheduling Irrigations: When and How Much. 1999. Water Management Series Publication 3396. Division of Agriculture and Natural Resources. University of California.
Basic Irrigation Scheduling in Florida. 1997. Bulletin 249. University of Florida Cooperative Extension Service.
Tensiometer Use in Irrigation Scheduling, L-797. 1997. Kansas State University Cooperative Extension Service.
Scheduling Irrigations by Electrical Resistance Blocks, L-901. 1994. Kansas State University Cooperative Extension Service.
Phillip Kaspari, Extension Faculty, Agriculture and Horticulture. Originally prepared by Peter Bierman, former Extension Faculty, Agriculture and Horticulture.
Reviewed October 2018